How to create things that don’t exist

We spoke to the Department of Applied Physics’ newest assistant professor Jose Lado, who’s joined Aalto University from ETH Zürich, about his research and what motivates him in his work.
What do you study, and why?

I do theoretical physics, and specifically the physics of what unconventional things we can make out of the electrons in materials. The branch of physics I’m interested in is called condensed matter physics, and one of the things we examine is how electrons behave when we change their energy levels, which we call electron excitations. We can make exotic states that can’t exist in individual atoms and electrons, by exploiting what is called emergent collective phenomena. These emergent states can give rise to exotic electronic excitations that provide a platform to explore physics beyond what we found so far, and that ultimately in the future may open a new venue for quantum computing.
A material I am especially interested in is graphene, a two-dimensional sheet of carbon. My theoretical research is working out what we need to do to graphene – like what other materials should we combine it with or how we should combine different graphene layers – to be able to make completely new exotic excitations that otherwise don’t exist. The remarkable feature of graphene is that by combining rotated layers one can control not only how electrons move, but also how the interact, something that is nearly impossible to control in other materials. This opens many exciting possibilities, as creating some exotic states of matter requires controlling electronic interactions, that we can do now in graphene. There are many things that we can explore in this way, such as understanding how we could create a room temperature superconductor or how to create a material showing quantum properties at a macroscopic scale. For me though, an exciting objective would be creating particle that does not exist, and that has unique quantum, and potentially, ground breaking properties: a parafermion.
There’s already quite a lot of strong evidence that condensed matter physicists can make Majorana bound states in the lab on the surfaces of special hybrid semiconductor/superconductor materials. Majorana bound states behave like Majorana fermions, a particle that has only been theorized and has not been observed occurring naturally in the universe. This is important for scientists trying to build quantum computers because, Majorana bound states could be used to implement a special kind of quantum computing known as topological quantum computing.
However, theory shows that not all types of quantum algorithms can be implemented using Majorana bound states. Therefore, I’m interested in trying to work out how we can make a new kind of bound state, called parafermions. Like Majorana fermions, we don’t expect that we could “catch” one existing in the real world, we would have to make it as a bound state on the surface of an exotic material. But we do know that if we could make them in the lab, parafermions would allow us to carry out all types of quantum computing algorithms. As a result, in the long run a computer that worked using parafermions would potentially be able to implement any quantum algorithm, with potential ground-breaking impact, for example for computational drug design.
What interests you about your field?
I am interested in finding exotic things that can be construct theoretically and don’t exist in nature, but can only exist by combining ingredients in a clever way, it’s like solving a puzzle to create something that didn’t exist before. The problems I work on often combine three interesting things. Firstly, they are related to deep mathematical theories; secondly, these theories are applied to fundamental principles in physics; and thirdly this research could potentially have a large future impact on quantum technology. All three of these fields are exciting by themselves, and they are combined in the topics I am working on.
During my bachelor’s degree I did courses on theoretical physics and solid-state physics. I did a summer study project where I got more interested in emergent phenomena in condensed matter physics, inspiring me to do a master’s degree, and from there I did a PhD on emergent phenomena in graphene.
To be a researcher you have to be fascinated by a topic, you need to want to know more and try to get something that people haven't seen before. You shouldn't say "I'm fine with everything everyone knows" – instead you must challenge what is considered possible, and you must want to find something that hasn't been explored before.
What do you expect from the future?
When I started my research, graphene was a material with many outstanding interesting properties, but it was not considered to be the best material to explore creating exotic electron excitations. This changed less than 2 years ago, when it was realized that by combining different graphene layers in a clever way one could explore strongly interacting physics in graphene with outstanding flexibility. This finding opened a whole new research area, and proved that exotic strongly interacting phenomena can be explored in a system just made of carbon atoms.
This story tells us that outstanding surprises arise in science, and when they do they can open a whole new world of possibilities, that we may have totally overlooked before. I find this very exciting, and it tells me that by looking in the right spot you may open a whole new world to explore. So a thing I would like to do is find out that another type of material or phenomena that is unexpectedly useful to explore exotic physical phenomena that we haven't thought about yet, and that we have so far overlooked. The thing most important to me in the future is to find something unexpected, which to me is the most exciting part of research.
Contact Details
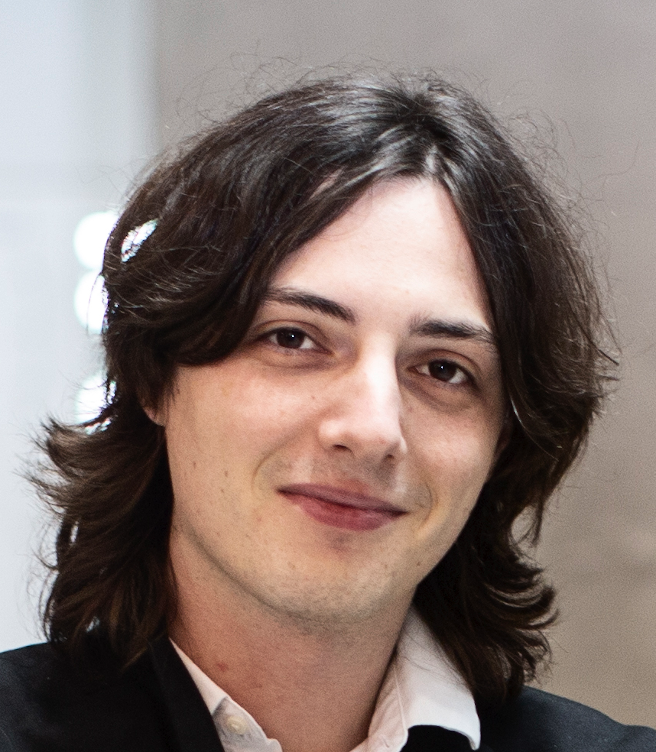
Related Stories
First confirmation of a Wigner crystal in graphene paves the way for a new kind of quantum computing
Using graphene, exotic arrangements of electrons can be studied, with possible applications including quantum computing.

Nanowires replace Newton’s famous glass prism
Researchers have designed the smallest ever spectrometer, which could turn a smartphone into a microscope

Correlated Quantum Materials (CQM)
Correlated Quantum Materials Group (CQM)
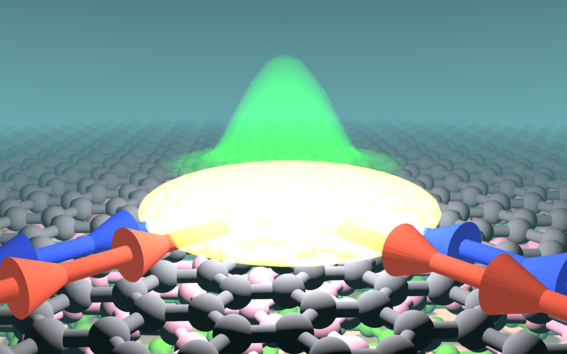
- Published:
- Updated:
Read more news

What makes nature restorative? Aalto University researchers explore Finnish forests and Japanese gardens
Biodiversity is central to the restorative power of Finnish forests.
New technology brings immersive audio to everyone’s pockets
A new type of sound recording technology allows recording of immersive soundscapes with ordinary microphones and an inexpensive accessory
Funding for a democratic transition to sustainability
Three projects from Aalto University are among the recipients. The Nessling Foundation's grants aim to advance the implementation of sustainability transitions in the context of democracy, the EU, and nature conservation areas.